Convection cooling is used in various devices, from mobile phone handsets to various consumer, communications, and industrial systems where the thermal loading is not too demanding. Devices such as heatsinks, heat spreaders, and heat pipes are used to implement convection cooling. Convection cooling systems are often classified as either passive or active.
Active cooling systems include fans, blowers, or pumps to move a cooling medium across the heatsink and improve the system’s thermal efficiency. In parts three and four of this FAQ series, active cooling systems will be discussed, which will consider “Forced air cooling keeps cool with fans and blowers” and “Liquid cooling for high-performance thermal management,” respectively.
Passive heat sinks have no moving parts and rely on pure convection to dissipate excess heat. They are more reliable but also larger than active systems. In addition, it’s important to provide continuous airflow and not block access to the airflow. Heatsinks can be classified by their structure, the material used in their manufacture, and the specific manufacturing process used to produce them.
Heat sinks are usually made from aluminum or copper. Each has its advantages. Aluminum is the most common material for heat sinks. In particular, extruded aluminum heat sinks fit the needs of most systems. Aluminum is relatively low cost, is lightweight, and has good thermal conductivity. Copper has better thermal conductivity than aluminum, but it is heavier and more expensive. Copper is used where the need for higher thermal conductivity outweighs other considerations. It is often found in active cooling systems. Heat sinks are also offered with various fin types, such as pin fins, straight fins, and flared fins.

In addition to distinguishing heat sinks based on the materials used and the fins’ structure, there are various manufacturing processes used to make heat sinks. The manufacturing processes are suited to specific materials and result in heat sinks with varying cost/performance tradeoffs:
Extruded Heat Sinks – The majority of heat sinks are made from extruded aluminum. The process is useful for most applications. Extruded heat sinks come at a low cost, and custom specifications can be easily manufactured. The performance of extruded heat sinks can range from low to high. The maximum width of extrusion limits Their dimensions.
Bonded Heat Sinks – These are normally used for applications that require larger heat sinks than can be made using extrusion. One benefit of these is that the base material and fin material can be different. Also, a combination of aluminum and copper fins can be used instead of just one fin material. This can support improved thermal performance while adding a minimal amount of weight. Bonded-fin heat sinks generally offer moderate performance at a higher cost.

Skived Heat Sinks – Heat sinks produced through this method are normally made from copper. They are made with a skiving machine that uses a blade to cut copper into thin slices and then push the skived fin up. For a heatsink application, the manufacture of ultra-thin fins is possible, as thin as 0.008”. The process allows high aspect ratios that provide greater surface area for maximum heat dissipation. These heatsinks offer medium to high performance, but their drawbacks are generally high weight and directional sensitivity.
Stamped Heat Sinks – In this process, metal fins are stamped and then soldered onto the base. These low-performance heat sinks are generally used for low-power applications. The advantage of stamped heat sinks is their very low cost due to ease of production automation.
Forged Heat Sinks – Forged heat sinks are made by compressing aluminum or copper and have many applications. Heat sinks can be cold forged or hot forged. These offer medium performance and can be made at a low cost for high volumes. However, they offer limited design flexibility.
Machined Heat Sinks – These are usually produced on a CNC machine and offer high thermal conductivity. Machining enables the production of complex geometries, which increases design flexibility. But they come at a high cost, and each piece’s production time makes them less-than-ideal for high volumes.
Graphite heat spreaders
Graphite heat spreaders are found in a wide variety of applications, including mobile phone handsets, tablet computers, OLED and LCD/LED displays, set-top boxes, electric vehicle batteries, grid storage batteries, automotive and transportation electronics, aerospace systems, and so on.
Graphite heat spreaders are lightweight heat spreading solutions that are available as simple sheets of material or in more complex assemblies with added mechanical strength. Heat spreaders can be beneficial components in a thermal management system as electronics increase in power and density, generating more heat. They utilize the high thermal conductivity of annealed pyrolytic graphite (APG) to spread heat evenly and quickly away from sensitive components within a sealed mechanical envelope. Since graphite is brittle and flaky, this envelope prevents graphite particles from breaking apart within a device, negating the inherent potential of brittle graphite flakes, causing a device to short or contaminating a clean environment.
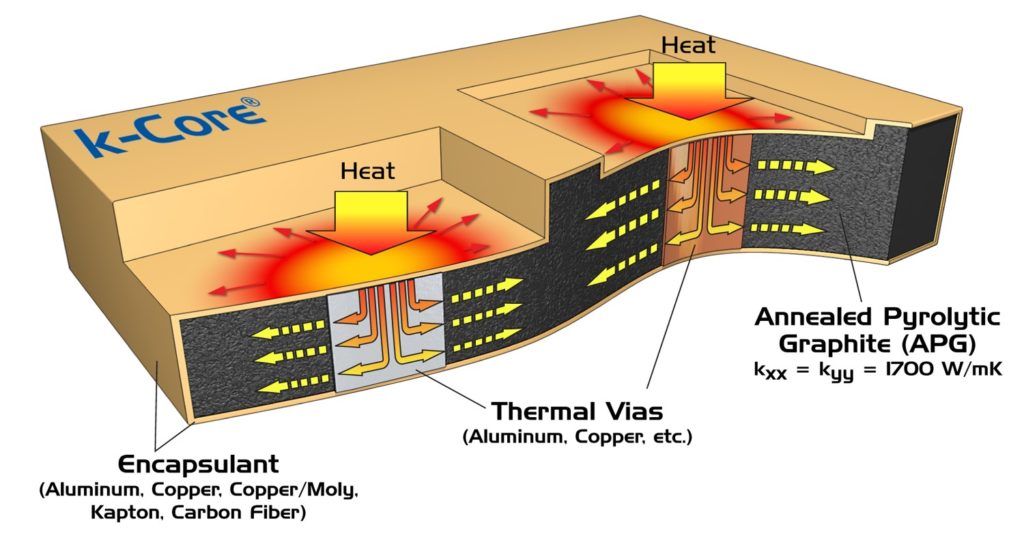
Polyimide films are a cost-effective alternative to traditional metal or ceramic encapsulants, making it suitable for high-volume applications. Since graphite is a lightweight, high conductivity material, encapsulated graphite heat spreaders offer a solution that can result in weight savings or smaller formats without sacrificing performance.
In addition to their heat spreading capacity, graphite heat spreaders can be integrated with other thermal management technologies, including finned structures and forced-air cooling. And an array of materials such as aluminum or copper alloys, ceramics, or composites can be used as the encapsulating material that enables thermal engineers to tailor the coefficients of thermal expansion (CTE) as needed.
Heat pipes
A heat pipe is a heat-transfer device that combines the principles of both thermal conductivity and phase transition to transfer heat between two solid interfaces effectively. Advantages of heat pipes include high thermal conductivity (10,000 to 100,000 W/m⋅K), isothermal operation, no moving parts, lower cost than most active cooling systems, shock- and vibration-tolerant, and freeze/thaw tolerant.
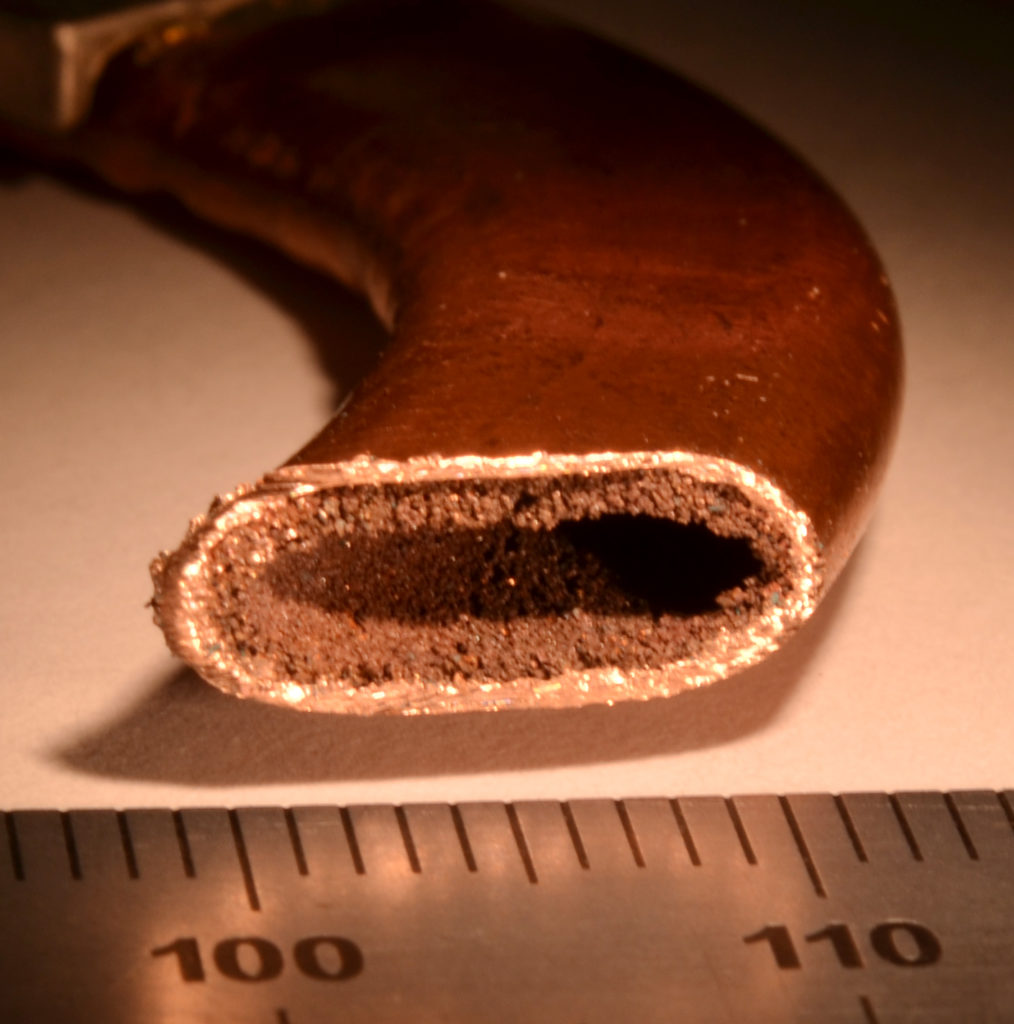
At the hot interface of a heat pipe, a liquid in contact with a thermally conductive solid surface becomes a vapor by absorbing heat from that surface. The vapor then travels along the heat pipe to the cold interface and condenses back into a liquid, releasing the latent heat. The liquid then returns to the hot interface through either capillary action, centrifugal force, or gravity, and the cycle repeats.
Due to the very high heat transfer coefficients for boiling and condensation, heat pipes are highly effective thermal conductors. The effective thermal conductivity varies with heat pipe length and can approach 100 kW/(m⋅K) for long heat pipes compared to approximately 0.4 kW/(m⋅K) for copper.
The most commonly used envelope/fluid pairs include:
- Copper envelope with water working fluid for electronics cooling. This is by far the most common type of heat pipe.
- Copper or steel envelope with refrigerant R134a working fluid for energy recovery in HVAC systems.
- Aluminum envelope with ammonia working fluid for spacecraft thermal control.
- Superalloy envelope with alkali metal (cesium, potassium, sodium) working fluid for high-temperature heat pipes is most commonly used to calibrate primary temperature measurement devices.
Heat pipes can support several specific thermal management use cases:
- Transfer of heat from one location to another. For example, many electronics use this to transfer heat from a chip to a remote heat sink.
- Transform heat from a high heat flux at the evaporator to a lower heat flux at the condenser, making it easier to remove overall heat with conventional liquid or air cooling methods. Heat fluxes of up to 1,000 W/cm2 can be transformed with custom vapor chambers.
- Provide an isothermal surface. Examples include operating multiple laser diodes at the same temperature and providing very isothermal surfaces for temperature calibration. An isothermal process is a type of thermodynamic process in which the system’s temperature remains constant: ΔT = 0. This typically occurs when a system is in contact with an outside thermal reservoir. The change in the system will occur slowly enough to allow the system to continue to adjust to the temperature of the reservoir through heat exchange.
This FAQ looked at the various options for convection cooling components. The remaining three FAQs in this series will dig into various methods and devices used to implement higher performance thermal management systems, including the use of fans and blowers to increase thermal transfer rates in part three and liquid cooling high-performance systems in part four.
References:
6 Heat Sink Types, Gabrian International
Encapsulated Graphite Heat Spreaders, Boyd Corp.
Heat pipe, Wikipedia
Heat sink, Wikipedia
Leave a Reply